Mini Review / Open Access
DOI: 10.31488/jjm.1000137
Cellular Effects Following Exposure to Mobile Phone Radiation and its Compensation
Peter C. Dartsch*1,Timo Dochow2
Dartsch Scientific GmbH, Institut für zellbiologische Testsysteme,49419 Wagenfeld, Germany
IfFP Privates Institut für feinstoffliche Forschung und Produktentwicklung GmbH,83026 Rosenheim, Germany
*Corresponding author:Prof. Dr. Peter C. Dartsch, Dartsch Scientific GmbH, Institute for Cell Biological Test Systems, Auf der Vosshardt 25, D-49419 Wagenfeld, Germany, Tel: +49 5444 980 1322;
Abstract
The effect of non-thermal radiation on biological systems is still discussed controversial. In this present study we investigated the non-thermal effects of an actively transmitting 1800 MHz mobile phone on cultured connective tissue fibroblasts(L-929). Cell vitality was examined morphologically and by using a colorimetric assay. For an exposure period of 2 h followed by a 22 h period of normal incubation, only 8.8 ± 6.1% (mean value ± standard deviation; n = 5) of the cells survived for total radiation with an intensity of 1.8W/m2at the level of the cells and 45.3 ± 5.3 % (mean value ± standard deviation;n = 13) of the cells survived for non-thermal radiation with an intensity of 1.25W/m2at the level of the cells. The exposure to non-thermal radiation was produced by placing a double wall corrugated board between the mobile phone and the cell layers. Cell vitality after exposure was independent from the mobile phone orientation, i.e. whether the display or the reverse side was directed towards the cells. In addition, we examined a device for its efficacy to compensate the non-thermal radiation of an actively transmitting mobile phone. At the same experimental conditions as mentioned above the device increased cell vitality to 82.4 ± 7.5 % (n = 6) or by the additional use of a specific mobile phone cover to 95.5 ± 6.1 % (n = 5). The use of an inactive dummy device caused no protective effect (n = 4). The results demonstrate that non-thermal radiation affects vitality of cultured connective tissue fibroblasts. The study also demonstrates that this specific device is able to compensate this radiation to a large extent (> 95 %).
Keywords: electromagnetic field; 1800 MHz; mobile phone radiation; non-thermal effect; oxidative stress; L-929; fibroblasts; cell death; cell culture
Introduction
Mobile data communication has become an integral part of everyday life. Today, nearly all technical devices work with mobile data transmission. Therefore, the electromagnetic tolerance of this technology is an important part of current research. In the next few years, the new 5G net-work will be implemented. The effect on humans remains still not clear to this day. In addition to the known thermal effects which are aimed at the warming of the body tissue, non-thermal effects also occur in biological systems and have already been discussed in several publications [1-5]. Although the mechanism of action of non-thermal effects continues to be controversial [6,7], there are some innovative theoretical approaches that require further investigation.
In 2011, the World Health Organization (WHO) classified high-frequency electromagnetic fields as potentially carcinogenic (2B) [8]. Since then, the application and expansion of high-frequency fields has increased dramatically and makes the relevance of past research very difficult when compared to current technological advances. The latest measuring methods in the areas of whole-body dosimetry and cell biology show the current status. Current standards of the IEEE and the ICNIRP [9,10] are still based on the thermal compatibility of biological organisms.
The main problem of studies of whole multi-cellular organisms such as rats, mice, drosophila and others is the complexity of the test systems. There are numerous unknown variables which are difficult to be established. In contrast to these models, cultivation of eukaryotic cells can be standardized and provides the opportunity to vary different factors depending on the experimental needs. Prompted by this background, we conducted a study in order to clarify whether high-frequency electromagnetic fields created by a commercially available mobile phone might cause non-thermal effects on cultured connective tissue cells. Moreover, we also studied the question whether a newly constructed device might be able to compensate the non-thermal effects of mobile phone radiation influencing biological systems, but not the thermal effects or the technical functionality.
Materials and Methods
Cell culture
In the present study, cultured connective tissue fibroblasts (cell line L-929; Leibniz-Institut, Deutsche SammlungfürMikroorganismen und Zellkulturen, Braunschweig, Germany) as a standard cell line for toxicological studies were taken at passages 22 to 50 over a total experimental period of 8 months. Cells were routinely cultivated in the moist atmosphere of an incubator at 37 °C and gassed with 5 % CO2 and 95 % air to yield a constant pH value. Culture medium was RPMI 1640 with 10 % fetal calf serum and standard amounts of gentamycin. All cell culture reagents were from Capricorn Scientific, 35085 Ebsdorfergrund, Germany.
Experimental design
For the tests, cells were seeded from 80 to 90 % confluent mass cultures at a density of 20,000 cells/well into 24 wells in the middle part of a 96 well-plate (200 µL culture medium/well). After 24 hours to ensure cell attachment and metabolisation, culture medium was exchanged to 200 µL/well of Leibowitz L-15 medium containing 10 % fetal calf serum and standard amounts of gentamycin. This culture medium guarantees a pH value at 7.4 even at normal atmospheric conditions. Plates were transferred to a Cultura M mini incubator and cultivated further at 37 ± 1 °C without CO2 gassing. A commercially available and actively transmitting 1800 MHz mobile phone (SAR value 1.510) at continuous operation mode was used to conduct the different test conditions. Radiation intensitieswere measured at the level of the cells at the same conditions used for the assays with an AaroniaSpectran HF-4060 equipped with a calibrated area antenna of 1 cm2. An intensity of 1.8W/m2 was measured for the actively transmitting mobile phone without an corrugated cardboard and 1.25 W/m2 when the same corrugated cardboard as used for the cell experiments was placed between the mobile phone and the cells.
In adition, the cardboard had also marked effects on the local temperature. When using the exposure times as in this study, the transmitting mobile caused a surface temperature at the level of the cells of approximately 46 °C, but when we used the cardboard we measured a value of 37.5 to 38 °C at the same position. So we concluded that our experimental design omitted thermal effects. The temperature in the incubator was keep constant at 37 ± 1 °C.
All tests were conducted with unexposed control cells in a 96-well plate at the same cultivation conditions, but approximately 10 meters distant from the active mobile phone in anCultura M mini incubator at 37 ± 1 °C without CO2 gassing. Cell vitality was checked by morphological observation of the cell cultures and by enzymatic activity. For this purpose, cell culture medium was removed and replaced by 180 µL fresh culture medium and 20 µL of XTT (Xenometrix AG, Allschwil, Switzerland) and incubated for 120 minutes in the incubator at 37 °C. XTT is the sodium salt of 2,3-bis[2-methoxy-4-nitro-5-sulfo-phenyl]-2H-tetrazolium-5-carboxyanilide and has a yellowish color. XTT is cleaved to an orange formazan by a complex cellular mechanism which occurs in viable cells only, and is related to NAD(P)H production by glycolysis. Therefore, the amount of formazan dye formed directly correlates to the number of metabolically active cells in the culture [11,12].
After 120 minutes, optical density was measured as a differential measurement ΔOD = 450 – 690 nm after a 4 second shaking interval using an ELISA reader (BioTek Slx808; Bad Friedrichshall; Germany).
Statistical analysis
Statistical analysis of all test assays was done using two-tailed Wilcoxon-Mann-Whitney test.
Compensatory device
A device was used to test its potential in compensating the effects of radiation exposure on connective tissue cell cultures. This device is sold commercially under the name memonizerMOBILE by the manufacturer (Memon bionic instruments GmbH, DE 83026 Rosenheim, Germany).
The function and method of action of the device has already been described in detail elsewhere [13] and will only be referenced here. In short, this device is a multi-layered metamaterial that is capable of compensating the non-thermal effects of radiation. Various phyllosilicates are used and have already proven their protective function against the influences of electromagnetic fields on biological systems in various experiments [14]. The device has dimensions of 35 x 13 x 0.25 mm (L x W x H) and is placed directly in the vicinity of the charging socket of the mobile phone or the antenna. Natural finite impulse response (FIR) frequencies are supported in living organisms by the resonance frequencies of the phyllosilicates in the infrared (IR), near infrared (NIR) and ultraviolet (UV) range. Artificial electromagnetic frequencies block these natural areas and can be compensated by corresponding resonances of the phyllosilicates [15-17].
Prior to the experiments with the memonizerMOBILE as presented here, we conducted a set of related experiments by using a conventional router as the source of non-thermal electromagnetic radiation ± a memonizerWLAN. In these experiments, an active and inactive version of the device was tested in a way that the experimentator did not know what kind of device he tested (blind study). In each experiment it was easily possible to identify the active device. Thus, we concluded that it seems to be not necessary in the experiments presented here to use the setup of a blind study furthermore. In addition, we also did some experiments with the memonizerMOBILE by using an inactive dummy in order to see if the material itself might influence the results.
Results
Effect of total vs. non-thermal mobile phone radiation
In order to evaluate the effect of thermal and non-thermal radiation on the cells, the actively transmitting mobile phone was directly placed on the lid of the culture plate without and with a double wall corrugated board (thickness: 7 mm) between mobile phone and cell layer to eliminate the influence of thermal effects by microwaves. Cells were exposed to mobile phone radiation as follows: 0.5 h of radiation exposure + 23.5 h of normal incubation; 1 h of radiation exposure + 23 h of normal incubation; 2 h of radiation exposure + 22 h of normal incubation; 4 h of radiation exposure + 20 h of normal incubation; 24 h of radiation exposure without additional normal incubation. The additional normal incubation period ensures that cellular repair might become active.
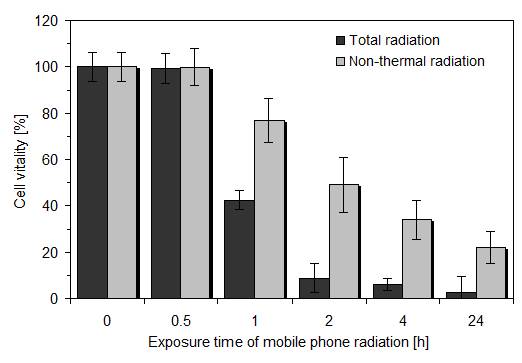
As depicted in Figure 1, the total effect of radiation on cell vitality was time-dependent when the actively transmitting mobile phone was directly placed on the lid of the culture plate. At exposure times ≥ 1 h, the reduced cell vitality was statistically significant when compared to untreated control and to the non-thermal radiation effect (p < 0.01; Wilcoxon- Mann-Whitney test). The ET50, i.e. the exposure time causing 50 % loss in cell vitality was calculated to be 0.75 h and cell vitality for 2 h exposure time (which was taken in further experimental approaches) was 8.8 ± 6.1 % (mean value ± standard deviation; n = 5). Examination of the non-thermal radiation effect by placing a double wall corrugated board between active mobile phone and cell layers also caused a time-dependent loss in cell vitality, but the time-vitality curve decreased much slower. Thus, the ET50 was calculated to be approximately 2 h and cell vitality for 2 h exposure time was 45.3 ± 5.3 % (mean value ± standard deviation; n = 13). The reduced cell vitality was also statistically significant (p < 0.01) at exposure times ≥ 1 h.
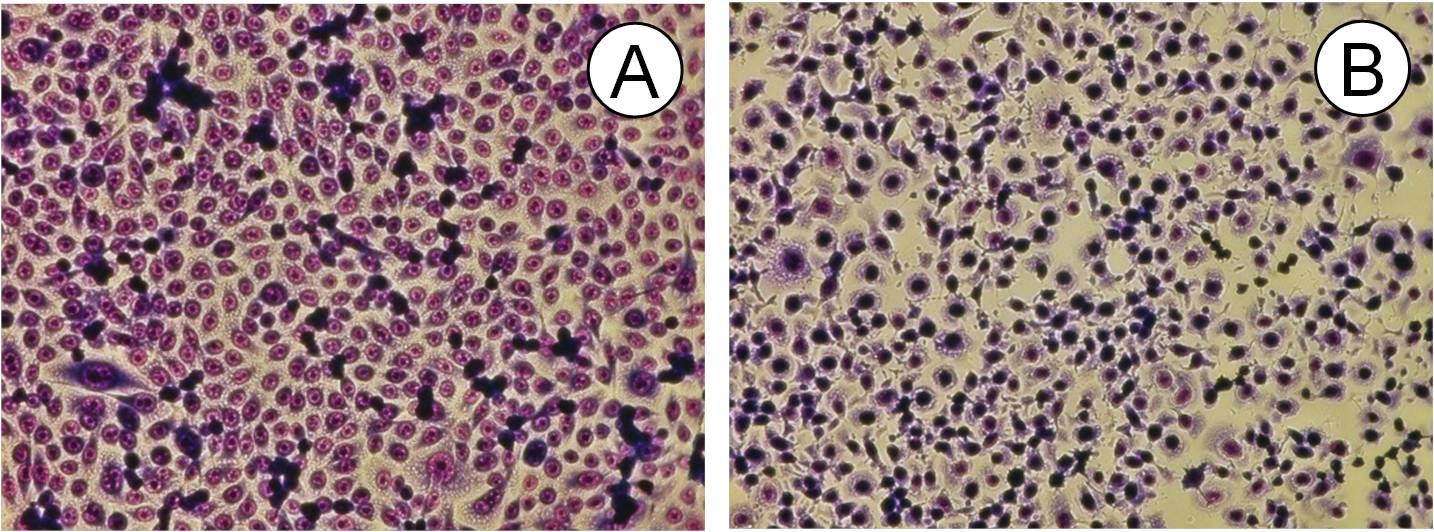
Morphological examination of exposed cells showed similar alterations in cell shape as already described for wireless DECT base radiation at 1.885 GHz [13] such as cell rounding, detachment and intracellular vacuolization which might be related to oxidative stress (Figure 2) which was also visualised by time-lapse video micrography (not depicted).
Effect of mobile phone orientation
Based on the results described in the last chapter, we used the same radiation and additional normal incubation parameters with the double wall corrugated board between active mobile phone and cell layers. However, in one experimental series the display of the mobile phone was placed towards the underlying cell layers and in a second series the reverse side of the mobile phone was directed towards the cells. The cause for these experiments was to examine whether the display which becomes quite hot when the mobile phone is actively transmitting, might also increase both, thermal and non-thermal radiation.
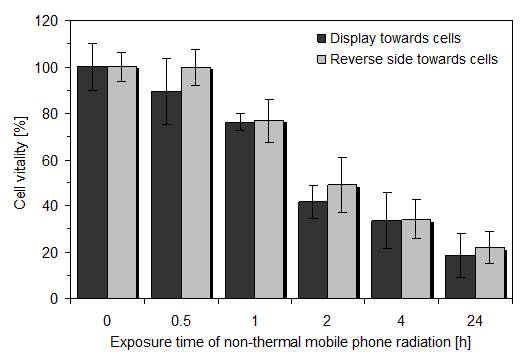
As shown in figure 3, there was no significant difference between both mobile phone orientations indicating that the display is mainly emitting microwaves and minor non-thermal radiation. Thus, for easier handling, the active mobile phone was always placed with its reverse side towards the cell layers in further experiments.
Efficacy of the device to compensate non-thermal mobile phone radiation
Based on the results described so far, we investigated whether the compensatory device is able to protect connective tissue cells from non-thermal mobile phone radiation. In all experimental series, cells were exposed to 2 h of the actively transmitting mobile phone followed by a 22 h period of normal incubation. A double wall corrugated board was placed between active mobile phone and cell layers and the mobile phone orientation was always with the reverse side towards the cell layers. The compensatory device was either placed on the rechargeable battery unit in the mobile phone or in the specially designed clamp of the mobile phone cover. In order to examine whether a protection might be due to the presence of the device material alone, an inactive dummy of the device was used to conduct the same experiments.
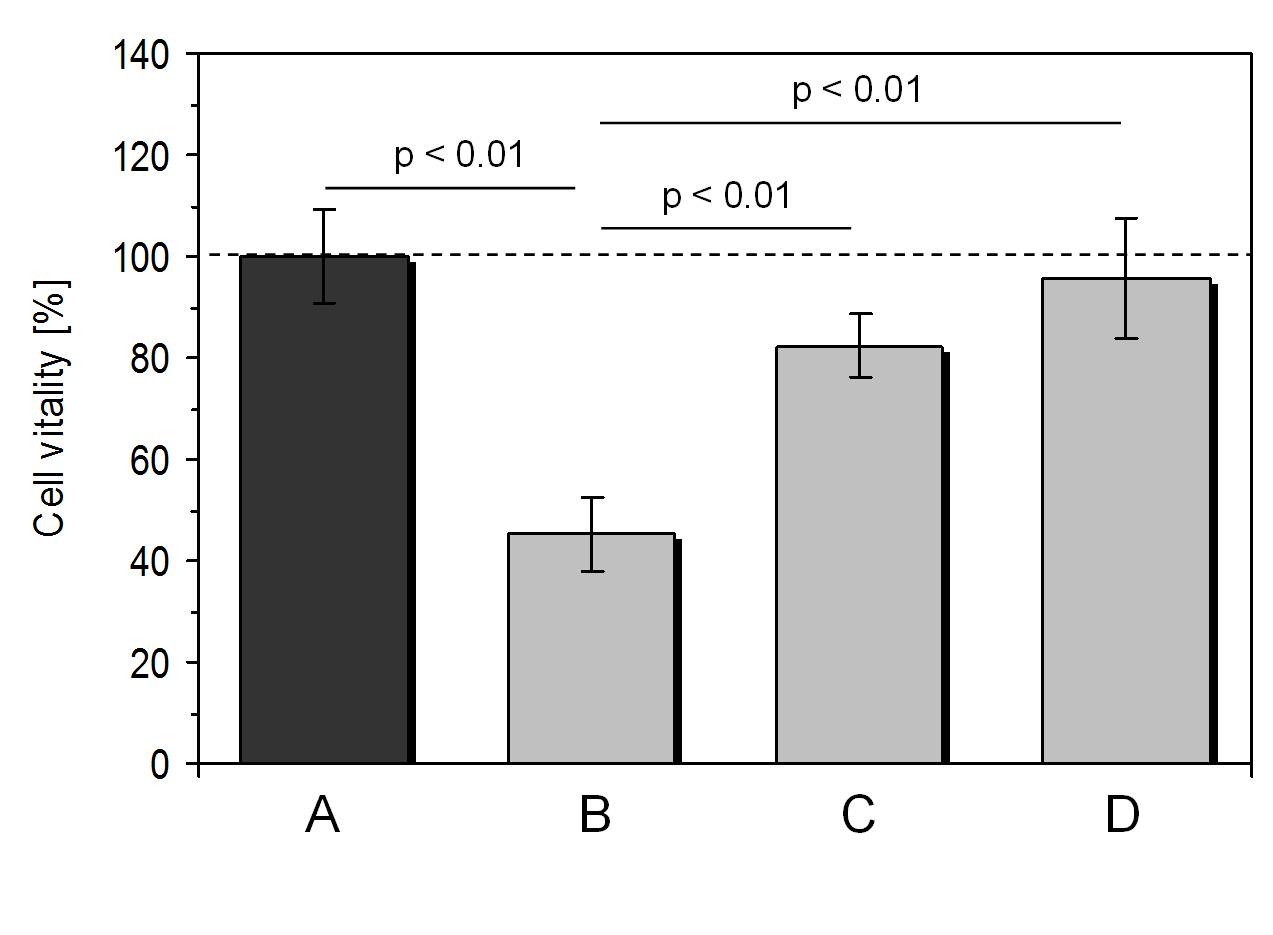
As depicted in Figure 4, the use of the compensatory device resulted in an enhanced cell vitality of 82.4%± 7.4% (mean value ± standard deviation; n = 6) in comparison to cells exposed to the actively transmitting mobile phone without the device (cell vitality: 45.3% ± 5.3%; mean value ± standard deviation; n = 13). The use of the compensatory device clamped in a specially designed mobile phone cover caused a cell vitality of 95.5% ± 6.1% (mean value ± standard deviation; n = 5) which means that this combination was able to compensate the non-thermal radiation of the actively transmitting mobile phone by a great extent which did not differ significantly from untreated controls.
Finally, we observed no significant difference between the effect of the non-thermal mobile phone radiation on cells without protection of the compensatory device (cell vitality: 45.3% ± 5.3%; mean value ± standard deviation; n = 13) or when using an inactive dummy of the device (cell vitality: 50.5% ± 7.6%; mean value ± standard deviation; n = 4).
Discussion
The first main question of the present study was to evaluate the effect of non-thermal 1800 MHz radiation of an active mobile phone in operation (talking) mode in relation to exposure time on cultured connective tissue fibroblasts as a sensitive biological test system. Our results showed that only 2 hours of continuous exposure to non-thermal radiation caused reduced cell vitality by more than 50 % in comparison to untreated control cells. The effect of the total radiation including the portion of microwaves caused more than 90 % loss in cell vitality. This effect is in accordance with other reports on the time-dependent effect of high-frequency radiation on cultured cells of different origin [18-21]. New in our study is the possibility to distinguish between the portion of thermal and non-thermal radiation by placing a double wall corrugated board between the actively transmitting mobile phone and the cell layers. The difference between both, total radiation and non-thermal radiation, is more than 40 % in cell vitality.
Somehow surprising was the result that cell vitality was not influenced by the orientation of the actively transmitting mobile phone. One should expect that the orientation with the display directed towards the cell layers, as in normal use of the mobile phone, would decrease cell vitality much more than the reverse side directed towards the cells. Lewicka et al. [22] investigated the changes taking place in human blood platelets under the effect of electromagnetic radiation emitted by LCD monitors. They found adverse effects within blood platelets’ oxygen metabolism which might cause physiological dysfunction of the organism. However, it is conceivable that most of the radiation emitted by displays is thermal which has been blocked in our test sytem by use of the cardboard.
Reactive oxygen and nitrogen species ubiquitously exist in complex biological systems, tissues or cells to participate in various cellular signaling pathways. They play an important homeostatic role in regulating the signal transduction involved in cell proliferation and cell survival [23]. Oxidative stress takes place when an excess of reactive oxygen and nitrogen species occurs. Then, endogenous detoxification of the reactive intermediates or repair of the oxidized biomolecules such as nucleic acids, lipids or proteins is not sufficient enough to avoid cell damage. Severe oxidative stress can cause cell death, and even a moderate action of oxidizing species can trigger apoptosis, while more intense stress may cause necrosis [24-27]. Cell death after electromagnetic radiation has been attributed in most publications to be related to increased levels of reactive oxygen and nitrogen species in a variety of species including human, tissues and cells (for review, see [28]).
Although the examination of oxidative stress itself was not a main point of interest in this study, we applied the subsequent normal incubation period after radiation exposure to allow the cells to regulate endogenous signaling for apoptotic or necrotic processes. As already described in detail by Kerr at al. [29] and El-Schich et al. [30], apoptosis shows characteristic morphological changes in single cells such as an increase in cell volume followed by a decrease and the formation of small, roughly spherical cytoplasmic fragments (apoptotic bodies). These typical signs were also observed in our study with cultivated connective tissue fibroblasts after mobile phone radiation suggesting that the cells also underwent apoptosis induced by oxidative stress as shown for cultivated fibroblasts [19,20]. Especially a time-lapse video demonstrated the initial increase in cell volume followed by shrinkage of the cells and the formation of apoptotic bodies after detachment (not shown here). The high pathogenic potential of the induced reactive oxygen species and their involvement in cell signaling pathways explains a wide range of biological and health effects of electromagnetic radiation which include both non-cancer and cancer pathologies [5,31-33].
The second main question of this study was to evaluate whether a special device might be able to compensate non-thermal mobile phone radiation during continuous operation. The results to this question are very convincing demonstrating that this device is actually able to compensate 1800 MHz non-thermal mobile phone radiation to a large extent without influencing the transmitted information. The efficacy of this device has been already shown in a previous publication [13].
It is planned to transfer the study settings to field experiments with humans in their normal living environment in order to see whether the results of the in vitro study as presented here can be assigned to a real life situation.
Conclusions
The results of our study demonstrate that exposure of cultured connective tissue cells to the non-thermal radiation of an actively transmitting mobile phone causes a significantly reduced cell vitality. However, this unwanted biological effect can be extensively compensated (> 95 %) by using the device either alone or with a specifically designed mobile phone cover.
Conflict of Interest
The authors declare no conflict of interests.
References
Funk RHW, Monsees TK. Effects of electromagnetic fields on cells: Physiological and therapeutical approaches and molecular mechanisms of interaction. Cells Tissues Organs.2006; 182:59–78.
Funk RHW, Monsees T, Özkucur N. Electromagnetic effects – from cell biology to medicine. ProgrHistochemCytochem. 2009; 43:177-264.
Giuliani L, Soffritti M. Non-thermal effects and mechanisms of interaction between electromagnetic fields and living matter. Eur J Oncol. 2010;
Yakymenko I, Sidorik E, Henshel D, et al. Low intensity radiofrequency radiation: A new oxidant for living cells. OxidAntioxid Med. 2014;Sci 3:1-3.
Yakymenko I, Tsybulin O, Sidorik E, et al. Oxidative mechanisms of biological activity of low-intensity radiofrequency radiation. ElectromagnBiol Med. 2016; 35:186-202.
Panagopoulos DJ, Johansson O, Carlo GL. Polarization: A key difference between man-made and natural electromagnetic fields, in regard to biological activity. Scientific Reports.2015; 5:14914.
Barnes F,Greenebaum B. Some effects of weak magnetic fields on biological systems – RF fields can change radical concentrations and cancer cell growth rates. IEEE Power Electronics Magazine.2016; 3:60-68.
Non-ionizing radiation Part 2: Radiofrequency electromagnetic fields. IARC Monogr.EvalCarcinog Risks Hum.2013; 102:1-460.
International Commission on Non-Ionizing Radiation Protection. Statement on the guidelines for limiting exposure to time-varying electric, magnetic, and electromagnetic fields (up to 300 GHz).Health Phys. 1998; 74:494-522.
International Commission on Non-Ionizing Radiation Protection. Statement on the guidelines for limiting exposure to time-varying electric, magnetic, and electromagnetic fields (up to 300 GHz). Health Phys. 2009; 97:257-258.
Roehm NW, Rodgers GH, Hatfield SM, et al. 19An improved colorimetric assay for cell proliferation and viability utilizing the tetrazolium salt XTT. J lmmunol Meth. 1991. 142:257-265.
Brosin A, Wolf V,Mattheus A,et al. Use of XTT-assay to assess the cytotoxicity of different surfactants and metal salts in human keratinocytes (HaCaT). A feasible method for in vitro testing of skin irritants.ActaDermatovenereologica. 77:26-28.
Dartsch PC,Dochow T. Cellular effects following exposure to wireless DECT base radiation and presentation of a device for their compensation. J Complement Alternat Med Res. 2017; 3:1-9.
Ferris JP. Mineral catalysis and prebiotic synthesis: Montmorillonite-catalyzed formation of RNA. Elements.2005; 1:145-149.
Avakyan SV, Serova AE, Voronin NA. The role of Rydberg atoms and molecules in the upper atmosphere. Geomagnetizm i Aéronomiya.1997; 37:99-106.
Sposito G, Skipper NT, Sutton R, et al. Surface geochemistry of the clay minerals. ProcNatlAcad Sci. 1999; 96:3358-3364.
Geesink HJH, Meijer DKF. Quantum wave information of life revealed: An algorithm for electromagnetic frequencies that create stability of biological order, with implications for brain function and consciousness. NeuroQuantol. 2016; 14:106-125.
Diem E, Schwarz C, Adlkofer F, et al. Non-thermal DNA breakage by mobile-phone radiation (1800 MHz) in human fibroblasts and in transformed GFSH-R17 rat granulosa cells in vitro. Mut Res/Genetic Toxicol Environ Mutagen. 2005; 583:178-183.
Manna D, Ghosh R. Effect of radiofrequency radiation in cultured mammalian cells: A review. ElectromagnBiol Med. 2016; 3:265-301.
Marjanovic CAM, Pavicic I, Lovakovic PB, et al.In vitro non-thermal oxidative stress response after 1800 MHz radiofrequency radiation. Gen PhysiolBiophys. 2017; 36:407-414.
Mahmoudi G, Nikzad S, Mehrpouyan M, et al. Effects of mobile phone prolonged radiation on kidney cells; an in-vitro study. J Renal Inj Prev. 2018; 7:175-179.
Lewicka M, Henrykowska GA, Pacholski K, et al. The effect of electromagnetic radiation emitted by display screens on cell oxygen metabolism – in vitro studies. Arch Med Sci. 2015; 11:1330-1339.
Finkel T. Signal transduction by reactive oxygen species. J Cell Biol. 2011; 194:7-15.
Ryter SW, Kim HP, Hoetzel A, et al. Mechanisms of cell death in oxidative stress. Antioxid Redox Signal. 2006; 9:49-89.
Franco R, Cidlowski JA. Apoptosis and glutathione: beyond an antioxidant. Cell Death Differ. 2009; 16:1303-1314.
Circu ML, Aw TY. Reactive oxygen species, cellular redox systems and apoptosis.Free RadicBiol Med. 2010; 48:749-762.
Navarro-Yepes J, Burns M, Anandhan A, et al. Oxidative stress, redox signaling, and autophagy: cell death versus survival. Antioxid Redox Signal. 2014; 21:66-85.
Wang H, Zhang X. Magnetic fields and reactive oxygen species. Int J Mol Sci. 2017; 18:2175-2195.
Kerr JFR, Wyllie AH, Currie AR. Apoptosis: A basic biological phenomenon with wideranging implications in tissue kinetics. Br J Cancer. 26:239-257.
El-Schich Z, Mölder A, Tassidis H, et al. Induction of morphological changes in death-induced cancer cells monitored by holographic microscopy.J Struct Biol. 2015; 189:207-212.
Hou Q, Wang M, Wu S, et al. Oxidative changes and apoptosis induced by 1800-MHz electromagnetic radiation in NIH/3T3 cells. ElectromagnBiol Med. 2015; 34:85-92.
Prasad M, Kathuria P, Nair P, et al. Mobile phone use and risk of brain tumours: a systematic review of association between study quality, source of funding, and research outcomes. Neurol Sci. 2017; 38:797–810.
Naziroglu M, Akman H. Effects of cellular phone- and Wi-Fi-induced electromagnetic radiation on oxidative stress and molecular pathways in brain. In: Systems Biology of Free Radicals and Antioxidants. Laher I (ed.); Springer; Berlin, Heidelberg.2014; 2431–2449.
Received: January 15, 2019;
Accepted: February 25, 2019;
Published: February 28, 2019.
To cite this article : Dartsch PC, Dochow T. Cellular Effects Following Exposure to Mobile Phone Radiation and its Compensation. Japan Journal of Medicine. 2019: 2:2.
©Dartsch PC, et al. 2019.